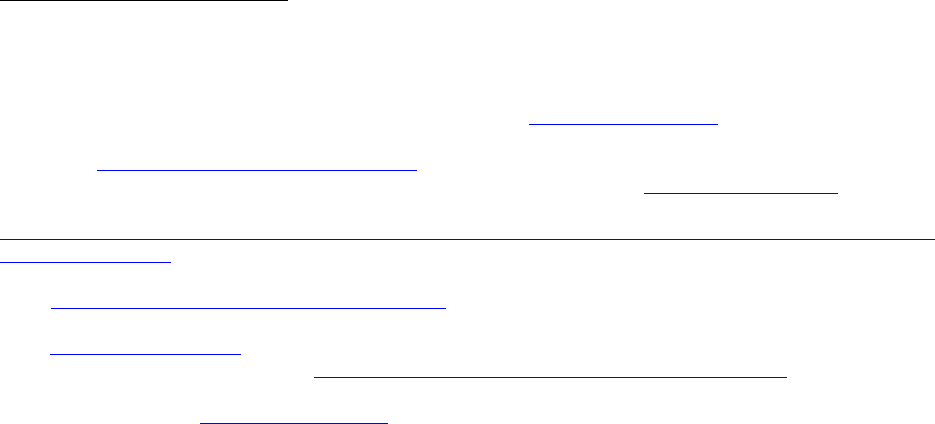
15
Charging Ahead with Batteries
The increase in EV sales in recent years has been enabled by the development of lower-cost
lithium-ion batteries supported by DOE research, which will continue to be a critical area going
forward. DOE models for EV battery fabrication costs have indicated that the expected cost of
EV batteries at high-volume has fallen by an astounding 70% since 2008.
55
The cost and performance of batteries are a key factor in continuing to lower the costs of EV
ownership. In addition to universities and industry, DOE has been a leader in battery R&D
investment: between 1992 and 2012, DOE invested $1 billion dollars in battery R&D, which
advanced the state-of-the-art by six years and created $3.5 billion worth of economic value.
56
This investment continues to pay off as rapidly falling costs mean that near-future battery costs
may be as low as $200/kWh by 2020.
57
Looking ahead, DOE is working with industry,
academia, and its national laboratories toward achieving an even more aggressive goal of
$125/kWh modeled production costs by 2022.
58
We can now see firsthand the results of advanced research in EV batteries making their way into
the market. For example, the energy storage capacity of the second-generation Chevrolet Volt
battery increased by 15% compared to the previous generation.
59
In general, optimization of cell
chemistry, design, and performance decrease the mass of battery packs, allowing EVs to travel
farther with full performance.
Road to the Future
Automakers are taking advantage of these innovations to design lower-priced EVs that are
poised to be strong competitors. For example, multiple automakers plan on delivering 200-mile-
range EVs for less than $40,000 around 2017.
60
As EVs accounted for 1.6% of all passenger cars
sold in 2014,
61
the potential for the next generation of EVs to impact transportation is large.
Improved charging infrastructure will also maintain momentum for EVs. There are now more
than 30,000 public and private EV charging outlets in the United States,
62
and as of August 2015,
55
Modeled costs are validated through applied research, and represent calculated high-volume commercial
production costs, rather than market price.
56
Inflation adjusted to 2014 dollars using U.S. Bureau of Economic Analysis GDP budget deflator. U.S. Department
of Energy. Benefit-Cost Evaluation of U.S. DOE Investment in Energy Storage Technologies for Hybrid and Electric
Cars and Trucks. Prepared by STI International. December 2013. http://go.usa.gov/3SnqJ
.
57
Nilson, M.; Nykvist, B.; Rapidly Falling Costs of Battery Packs for Electric Vehicles. Nature Climate Change,
April 2015. http://dx.doi.org/10.1038/nclimate2564
.
58
U.S. Department of Energy. EV Everywhere Grand Challenge. January 2014. http://go.usa.gov/3Sn3B.
59
“Chevrolet Introduces All-New 2016 Volt,” General Motors, January 12, 2015. Accessed Aug. 25, 2015:
http://media.gm.com/media/us/en/gm/news.detail.html/content/Pages/news/us/en/2015/Jan/naias/chevrolet/volt/0112
-volt-2016-intro.html.
60
Davies, A. “Chevy Could Beat Tesla to Building the First Mainstream Electric Car,” Wired. Accessed Aug. 21,
2015: http://www.wired.com/2015/01/chevrolet-bolt-ev
.
61
118,882 EVs sold in 2014 (Argonne National Laboratory. 2014 Vehicle Technologies Market Report. March
2015. http://go.usa.gov/3S735
) compared to 7,687,619 total passenger cars sold in 2014 (Ward’s Auto U.S. Car and
Truck Sales, 1931-2014. January 2015. http://wardsauto.com/keydata/historical/UsaSa01summary).
62
U.S. Department of Energy, Alternative Fuels Data Center. “Alternative Fueling Station Counts by State,”
Accessed October 2015. http://go.usa.gov/3S7rh
. Residential EVSE locations or “wall outlets” are not included.